Review Article - Journal of Drug and Alcohol Research ( 2024) Volume 13, Issue 11
Impact of Gut Dysbiosis on Neuromyelitis Optica Spectrum Disorder: Implications for Alcohol and Substance Use
Lourdes de Fatima Ibanez Valdes1 and Foyaca-Sibat Humberto2*2Department of Neurology, Walter Sisulu University, South Africa
Foyaca-Sibat Humberto, Department of Neurology, Walter Sisulu University, South Africa, Email: humbertofoyacasibat@gmail.com
Received: 30-Oct-2024, Manuscript No. JDAR-24-151653; Editor assigned: 01-Nov-2024, Pre QC No. JDAR-24-151653 (PQ); Reviewed: 15-Nov-2024, QC No. JDAR-24-151653; Revised: 20-Nov-2024, Manuscript No. JDAR-24-151653 (R); Published: 27-Nov-2024, DOI: 10.4303/JDAR/236418
Abstract
The Central Nervous System (CNS) is affected by the chronic inflammatory autoimmune disease known as Neuromyelitis Optica Spectrum Disorder (NMOSD). Acute myelitis, area postrema syndrome, acute brainstem syndrome, Optic Neuritis (ON), and symptomatic cerebral syndrome are among the primary clinical symptoms of NMOSD. People with NMOSD may experience significant visual impairments, mobility difficulties, and even death. With a 9:1 female to male ratio, the prevalence of NMOSD varies from 0.5 to 4 afflicted per 100,000.
NMOSD causes Astrocyte (As) and Oligodendrocyte (OLG) damage leading to demyelination and neurodegeneration. It typically does affect the optic nerves and the spinal cord, especially in long segments, but other features are helpful for the diagnosis. We review the relationship between NMOSD and dysbiosis as a main aid of this review.
Implications for alcohol and drug abuse in NMOSD: The therapy of NMOSD may become even more challenging if substance use, including alcohol, worsens gut dysbiosis and compromises immune function. Drinking alcohol can cause a heightened inflammatory response, increase intestinal permeability, and damage the integrity of the gut lining. The dysbiosis that already exists in NMOSD patients may be exacerbated by this disturbance in gut health, which could lead to increased symptoms and more relapses. Other medications, including some narcotics and stimulants, can potentially alter the gut microbiota’s composition and damage the central nervous system, which may have an adverse effect on the underlying immunological dysfunction in NMOSD. Dysbiosis and NMOSD are related, which emphasizes the need to treat substance use in NMOSD patients with caution because these conditions may affect gut health and perhaps exacerbate illness consequence Because dysbiosis and NMOSD are linked, it is important to treat substance use in NMOSD patients carefully because these disorders may impact gut health and perhaps worsen the effects of the illness. To help stabilize gut health and immunological function, more study on gut microbiota and its modulation may provide pharmacological targets for managing NMOSD and highlight lifestyle changes like cutting back on alcohol and other drugs.
Keywords
Neuromyelitis optica spectrum disorder; Alcohol; Drug; Transverse-longitudinal myelitis; Optic neuritis; Antibody-mediated disease; Astrocitopathy; Demyelinating disorder; Pityriasis versicolor; Dysbiosis; Gut Microbiota
List of Abbreviations
(AC) Anterior Chamber; (APS) Area Postrema Syndrome; (AQP-4) Aquaporin-4; (As) Astrocyte; (AZA) Azathioprine; (BBB) Blood-brain Barrier; (BMI) Body Mass Index; (CDR) Cup Disc Ratio; (CN) Cranial Nerves; (CNS) Central Nervous System; (CSF) Cerebrospinal Fluid; (CT) Computed Tomography; (EAAT 2) Excitatory Amino Acid Transporter 2; (ESR) Erythrocyte Sedimentation Rate; (FBC) Full Blood Count; (FC) Finger Counting; (HDAC) Histone Deacetylases; (HIV) Human Immunodeficiency Virus; (IL-6) Interleukin Six; (IV) Intravenous; (LETM) Longitudinally Extensive Transverse Myelitis; (LFT) Liver Function Tests; (LL) Lower Limbs; (MMF) Mycophenolate Mofetil; (MOG) Myelin Oligodendrocyte Glycoprotein; (MRI) Magnetic Resonance Imaging; (MS) Multiple Sclerosis; (NI) Neuroinflammation; (NLP) No Light Perception; (NMO) Neuromyelitis Optica; (NMOIgG) Neuromyelitis Optica Immunoglobulin G; (NMOSD) Neuromyelitis Optica Spectrum Disorder; (OCT) Ocular Cohesive Tomography; (OD) Ocular Dextro; (ON) Optic Neuritis; (OLG) Oligodendrocyte; (OS) Ocular Sinister; (OXPHOS) Oxidative Phosphorylation; (RORγ) Retinoic Acid Receptor-related Orphan Receptor gamma; (RTX) Rituximab; (STAT 3) Signal Transducer and Activator of Transcription 3; (SLE) Systemic Lupus Erythematosus; (UL) Upper Limbs; (VA) Visual Acuity
Introduction
The chronic inflammatory and immunological condition known as Neuromyelitis Optica Spectrum Disorder (NMOSD) affects the central nervous system. Acute myelitis, area postrema syndrome, acute brainstem syndrome, Optic Neuritis (ON), and symptomatic cerebral syndrome are among the primary clinical symptoms of NMOSD [1]. People with NMOSD may experience significant vision impairments, mobility difficulties, and even death [2]. With a 9:1 female to male ratio, the prevalence of NMOSD varies between 0.5 and 4 afflicted per 100,000 [3].
The diagnosis of NMOSD has relied heavily on the identification of Immunoglobulin G (IgG) antibodies against the Aquaporin 4 (AQP4) water channel in NMOSD patients [4]. In the brain, spinal cord, and optic nerves, AQP4 is the most extensively expressed bidirectional, osmotically driven water channel [5]. Localized to the peduncles of astrocytes at the Blood-brain Barrier (BBB), AQP4 is found in regions of the brain that come into contact with cerebrospinal fluid [6]. Even though AQP4 was determined to be the key target, it is still unclear what pathophysiological processes initially caused NMOSD to emerge. In addition to genetic factors, AQP4-antibodies (Ab) can also be triggered by environmental stimuli including bacteria, viruses, or plants [7,8]. However, other studies have demonstrated that T lymphocytes that recognize AQP4 epitopes cross-react with commensal bacteria’s homologous peptide sequences that are present in the human gut microbiota [9]. As a result, the role of gut microbiota as a novel risk factor has also been extensively researched recently.
NMOSD is the term given to a very uncommon autoimmune disorder characterized by demyelination and inflammation of the CNS. Typically, affecting the optic nerves and the spinal cord, causing myelitis and optic neuritis, respectively. Current advances have led us to appreciate that the clinical manifestations are much more extensive and include the brainstem and other regions in addition to those already mentioned.
While NMOSD does occur globally, it has an elevated prevalence among blacks, Asians, and Caucasians in descending order.
This interaction is further complicated by alcohol and substance use because of their well-known impacts on immune function and gut microbiome. For instance, it has been demonstrated that long-term alcohol use alters the composition of the gut microbiota, increases intestinal permeability, and stimulates systemic inflammation. Substance abuse can also change immunological responses and gut microbiota, which can exacerbate autoimmune diseases and dysbiosis. Understanding how alcohol and drug use may affect NMOSD through mechanisms involving gut dysbiosis is essential given these shared pathways.
Despite much research, the aetiology of NMOSD is still unclear; however, recent investigations on immunopathogenesis revealed the antibodies Aquaporin-4 (AQP-4) and Myelin Oligodendrocyte Glycoprotein, which contributed to clarifying the pathophysiology and thus managing this spectrum disease [1-3].
There are reported cases of NMOSD with other autoimmune systemic diseases such as Sjogren syndrome, Systemic Lupus Erythematosus, rare cutaneous manifestations and even hypertension [4-6].
The composition of the GM includes more than 1200 types of bacteria on top of other microorganisms such as viruses, fungi, archaea, the Gram-positive Firmicutes and the Gram-negative Bacteroides, the commonest ones, in healthy people. This dynamically balanced symbiotic relationship between the GM and the host plays a crucial role in human metabolism and immunity, and its disbalance causes Neuroimmunological (Nim) disorders and Neuroinflammation (NI). Below, we will review the correlation between NMOSD and dysbiosis as the main aim of this study.
The purpose of this study is to investigate the connection between gut dysbiosis and NMOSD, with an emphasis on how substance and alcohol abuse may alter this association and exacerbate symptoms. New treatment ideas and approaches for treating NMOSD in patients with a history of alcohol or drug use may result from an understanding of these relationships.
Brief Comments and Concluding Remarks
Neuromyelitis Optica (NMO) was reported to the medical literature by Allbutt et al. in 1870 [7]. NMOSD show a characteristic pattern of As dysfunction and cell loss, leading to neurodegeneration and demyelination [8-14].
Usually, it is followed up by a poor prognosis, and the disease is thought initially to be monophasic. As we and other investigators documented previously, NMOSD is an uncommon, chronic inflammatory autoimmune and disabling disease of the brain and spinal cord, including the optic nerve which it’s a prolongation of the brain, where aquaporin-4 (AQP4)-specific T lymphocytes play a vital role in its pathogenesis [15-25].
A typical lesion found at the beginning of the optic nerve in the retina and in the optic nerve can be seen in Figures 1 and 2.
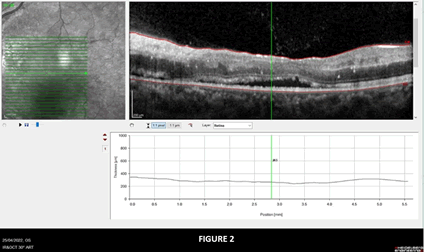
Figure 1: MRI T2W_3D_SPIR Optic nerve images showing signs of optic neuritis more evident on the side of the white arrow
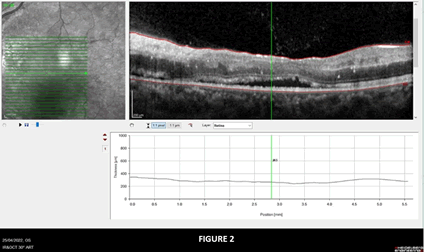
Figure 2: Shows OCT image of OS showing sub retinal fluid collection and exudates
In conclusion, the increasing evidence linking gut dysbiosis to Neuromyelitis Optica Spectrum Disorder (NMOSD) highlights the significance of gut health for Central Nervous System (CNS) autoimmune diseases. For NMOSD patients, maintaining a balanced gut microbiota may be crucial to managing their symptoms and slowing the disease’s progression. Furthermore, the effects of sickness may be worsened by lifestyle factors including substance addiction and alcohol intake that worsen intestinal dysbiosis and inflammation. Therefore, addressing these lifestyle factors should be a major part of NMOSD therapy regimens.
Other authors have demonstrated that T cells that recognize the AQP4 epitope exhibit cross-reactivity with homologous peptide sequences in certain microorganisms, including Clostridium perfringens, in addition to immune factors, corroborating the claim that GM is a key contributor to the pathogenicity of NMOSD [25].
We considered the role of dysbalanced GM (Dysbiosis) in other neurological conditions and documented it in previous publications. Based on those articles and other recent publications by Yao et al., we hypothesized that metabolites produced by dysbiosis, like tryptophan, bile acid, and Short-chain Fatty Acids (SCFAs), affect immune cell metabolism, modulating the pathophysiology of NMOSD as we graphically represented in Figures 3 and 4.
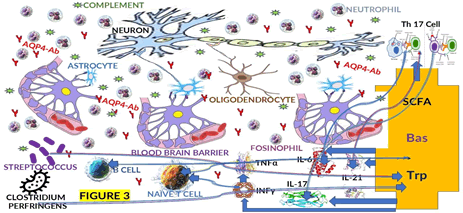
Figure 3: The pathophysiology of NMOSD is based on a graphic hypothesis that the GM (Streptococcus and Clostridium perfringens) has caused the immune cell metabolism to be affected by Small Chain Fatty Acids (SCFAs), Bile Acid (Bas), and Tryptophan (Trp). Proinflammatory cytokines such as TNF (Tumor Necrosis Factor) alpha, INF (Interferon) gamma, IL (Interleukin)-6/17/21, AQP4 (Aquaphorin-4)-Ab (Antibody), and Th (T helper) 17 cell are among the factors that modulate the pathophysiology of NMOSD
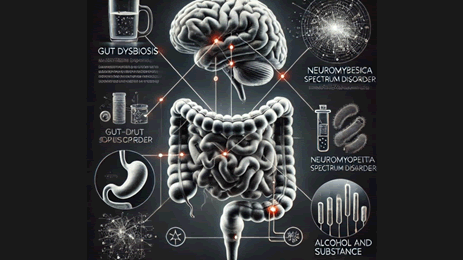
Figure 4: Impact of gut dysbiosis on neuromyelitis optica spectrum disorder: Role of alcohol and substance use
GM is primarily given by the mother at birth, and it develops further through interaction with changes in the outside world and the feeding process. The type of diet is the significant factor influencing changes in GM. According to the diet consumed, certain bacteria thrive in the human intestine. Nevertheless, the different diet components, such as proteins, fats, carbohydrates, vitamins and fibres, will change the composition of the GM [23-25].
Before this study, We hypothesized how aging and alcoholism impact GM’s composition, as well as how certain degenerative diseases and brain parasite conditions, such as neurocysticercosis, impact GM on the development and maturation of the mucosal immune system, the synthesis of antimicrobial and bacteriostatic substances, the regulation of gut neuromuscular function, the preservation and integrity of the gut barrier, and the safe execution of numerous vital metabolic processes, such as serving as a detoxifier and catalyzing the metabolism of drugs, hormones, and carcinogens, the effects on physiological systems, including the central nervous system, and lowering histamine synthesis [24]. In addition to altered gene expression linked to neurotransmission changes on the BBB permeability, we hypothesized that dysbiosis of the GM can result in changes in metabolites and microbiotaderived components. This dysregulation of the immune system can cause a variety of neurological conditions and injuries during neurodevelopmental plasticity, including neurodegenerative, neurodevelopmental disorders, and NMOSD.
According to immunological theory, astrocytes-AQP4 are the target cells in NMOSD instances, and antibodies to the NMO-IgG target conformation result in both AQP4-Ab and the extracellular loop of AQP4, which are expressed by damage or As destruction. We postulated that AQP4-Ab may cause As to produce Interleukin 6 (IL-6), and that IL-6 signaling reduces BBB function, resulting in autoimmune responses in the central nervous system. This hypothesis was based on documented reports of CNS injuries in cases presenting NMOSD, which are characterized by rosettelike deposits of IgG, IgM, and complement, primarily in the perivascular area [25].
AQP4-Ab has previously been shown to breach the bloodbrain barrier by binding to the extracellular AQP4 receptor, which expresses the conventional complement cascade. This is followed by the infiltration of T lymphocytes, granulocytes, and macrophages/microglia [26]. Neurons and supporting cells lose support as a result of the damage done to As. Demyelination, neurodegeneration, and neuronal loss with necrosis, cavities, vascular thickening, and hyalinization are caused by damaged Oligodendrocyte Precursor Cells (OPC)/Oligodendrocytes (Od) [26].
The T lymphocyte, B lymphocytes, endogenous, exogenous, and plasma cells are involved in the immune cascade response in the pathogenesis of NMOSD (Figure 3).
Gut dysbiosis, a microbial imbalance in the gastrointestinal tract that can result in a number of health problems, is highlighted in this graphic, which emphasizes the complex interaction between the gut and brain. The relationships between gut health and neurological conditions like neuromuscular spectrum disease and neuromodulation spectrum disorder are at the center of this graphic. The representation of every digestive system part, including the stomach and intestines, shows the ways in which gut health can affect mental health. Furthermore, elements like substance abuse and alcohol usage are shown, highlighting how they exacerbate gut dysbiosis and associated conditions. It is essential to comprehend these links in order to create comprehensive treatment plans that take brain and gastrointestinal health into account.
Gut Microbiota Associated with NMOSD
Clostridium perfringens
Apart from the Firmicutes/Bacteroidetes population, we hypothesized that Clostridium perfringens located in the normal gut is also considered a conditional pathogen in several contexts, including patients presenting NMOSD, as has been documented by other investigators [26-28].
Other authors also supported the idea that Clostridium perfringens is involved in the pathogenesis of NMOSD [29].
Markovich-Plese et al. found that AQP4-specific T-cell epitopes contain residues with 90% homology to sequences within the ATP Binding Cassette Transporter Permease (ABC-TP peptide of Clostridium perfringens), which supports the previous statement [30].
It is associated with the differentiation of pro-inflammatory Th17 cells, modulating the balance between proinflammatory and anti-inflammatory T cell subsets and damaging the neurons and supporting cells after passing through the BBB.
Other Gram-positive bacteria flora, like Streptococcus, can disrupt the epithelial cell integrity, mainly the cytoplasmic integrity, severely damage the mucosal skin resistance, and cause inflammation and its overrepresentation in cases with NMOSD have been reported by many authors [31-35].
Other investigators have reported higher levels of other GMs, such as Butyricimonas, Rothia, Veillonela, Haemophilus, and Alistipes [36].
An overview of the articles discussed in this section is presented in Table 1.
Table 1: An overview of studies investigating the role of GM in NMOSD
Samples | Number of NMO SD Patients | Gut microbiota | Results |
---|---|---|---|
Peripheral blood T cells | 18 | Clostridium perfringens | AQP4-specific T cell epitopes were found to contain residues with 90% homology to sequences within the ABC-TP. |
Th17 cells recognized immunodominant AQP4 epitopes and proliferated in response to the corresponding ABC-TP peptide. | |||
Fecal samples | 19 | Clostridium perfringens | Clostridium perfringens is the species most significantly enriched in NMOSD patients. |
Fecal and peripheral blood samples | 43 | Clostridium boltae | The prevalence of Clostridium boltae was significantly higher in AQP4-positive patients. |
In AQP4-positive NMOSD patients, Clostridium boltae was associated with inflammatory genes. |
We considered the role of dysbalanced GM (Dysbiosis) in other neurological conditions and documented it in previous publications. Based on those articles and other recent publications by Yao et al., We hypothesized that the pathophysiology of NMOSD is modulated by metabolites generated by dysbiosis, such as tryptophan, bile acid, and Short-chain Fatty Acids (SCFAs), which impact immune cell metabolism.
GM is mainly received from the mother at birth and progresses its development through contact with the external environment changes and feeding process. The type of diet is the significant factor influencing changes in GM. According to the diet consumed, certain bacteria thrive in the human intestine. Nevertheless, the different diet components, such as proteins, fats, carbohydrates, vitamins and fibres, will change the composition of the GM [23-25].
Discussion
Before this study, we speculated on the role of ageing and alcohol disorder in the composition of GM and the presence of some degenerative diseases and brain parasitic diseases like neurocysticercosis and the role of GM on the maturation and development the mucosal immune system, the production of antimicrobial and bacteriostatic substances, regulation of the gut neuromuscular function, the maintenance and integrity of the gut barrier and the healthy performance of many critical metabolic functions acting as a detoxifier and catalyzing the metabolism of drugs, hormones and carcinogens, the effects on physiological systems including the CNS, reducing histamine synthesis [24]. We hypothesized that dysbiosis of the GM can lead to modifications in metabolites and microbiota-derived components, as altered gene expression related to neurotransmission changes on the BBB permeability, leading to dysregulation of the immune system causing several types of neurological conditions and injuries during neurodevelopmental plasticity leading to neurodegenerative, neurodevelopmental disorders and NMOSD as well. From the immunological point of view, the target cells in cases of NMOSD are the astrocytes-AQP4 and the antibodies to NMO-IgG target conformational leads to both AQP4-Ab and the extracellular loop of AQP4, with complement, expressed by injury or destruction of the As. Based on the documented reports on the CNS injuries in cases presenting NMOSD characterized by rosette-like deposits of IgG, IgM, and complement, mainly in the perivascular area, we hypothesized that AQP4-Ab might trigger Interleukin 6 (IL-6) production by As and that IL-6 signalling diminish BBB function causing autoimmune responses in the CNS. We documented before that AQP4- Ab pass across the BBB binding to the extracellular AQP4 receptor, which expresses the classical complement cascade, which is followed by the infiltration of macrophages/ microglia, granulocytes, and T lymphocyte infiltration [25,26]. The damage caused to As leads to loss of support to neurons and supporting cells. Damaged Oligodendrocyte Precursor Cells (OPC)/Oligodendrocytes (Od) lead to demyelination, neurodegeneration, and neuronal loss with necrosis, cavities, vascular thickening and hyalinization [26].
The T lymphocyte, B lymphocytes, endogenous, exogenous, and plasma cells are involved in the immune cascade response in the pathogenesis of NMOSD.
In patients with dysbiosis, NCC, and AUD, a defective gut barrier links immune activation and dysbiosis, causing Mg polarization, which increases pro-inflammatory cytokines production, leading to a systemic inflammatory response, dysfunctional BBB, and NI, promoting neurodegeneration and consequent neurological damage [24]. Based on the previous statement, we hypothesized a similar mechanism in cases of NMOSD.
The idea of the “Enteric Neuroimmune Cell Unit” (ENCU) was developed in order to advance knowledge of the interaction between the immune system and the central nervous system. The ENCU is an internal sensory organ in charge of safeguarding and maintaining the function and integrity of the intestinal tract, enabling innate defence and memory responses against some diseases. Through commensal bacteria colonizing the GM and addressing the reciprocal signaling between tissue-resident immune cells and neurons/supporting cells, the ENCU develops and promotes the maturation of the enteric neuroimmune system [25]. The GM-brain axis, which is a complex network of communication between the GM and the CNS in the control of immunological, gastrointestinal, and neurological system functions via numerous different signaling pathways in the GBA, is what drives this communication between the brain and the GM, such as neural, HPA, endocrine, and immune signalling facilitated by the synthesis and release of many molecules, including cytokines, neuropeptides, neurotransmitters, and microbial metabolites as we documented several times [23].
However, at this point, we have yet to determine the mechanism by which the GM modulates the host neuroimmune homeostasis despite some authors highlighting
1. The effects of drastically lowered Short-chain Fatty Acids (SCFAs-acetate/butyrate [IFN-γ]) on the mucosa’s immune response, including decreased BBB permeability, Oxidative Phosphorylation (OXPHOS) Stimulation, G protein-coupled receptor activation, inhibition of Histone Deacetylase (HDAC) 40, HDAC activity inhibition, and increased antibody production;
2. Tryptophan (Trp) Food intake (dietary proteins) provides the gut microbiota with tryptophan catabolic metabolites, which use the aryl hydrocarbon receptor (AhR) expression pathway to decrease Th17 cell numbers and downregulate RORγ (Retinoic Acid Receptor-related Orphan Receptor gamma) and STAT3 (Signal Transducer and Activator of Transcription
3. There are also many reports on the role of GM-derived tryptophan in B cells [25].
However, we do not know how GM-derived tryptophan directly regulates B cell IL-10 production as antibody production. Bile Acids (BAs): Additionally, BA metabolites interfere with intracellular calcium homeostasis, which is essential for T lymphocyte production and NFAT (Nuclear Factor for Activating T Cells) signalling [37]. The BA metabolite induces the PCD of activated B cells and blocks Ig production. Numerous investigations have demonstrated that in order to induce autoimmune demyelination, the GM was required to initiate myelin autoantigen recognition. NF-κB, the main regulator of pro-inflammatory reactions, is suppressed when it is activated [37]. All 3 metabolites act on immune cell expression and differentiation, as we graphically represent in Figure 1. We hypothesized in earlier research that a compromised immune system would encourage naive T cell transformation into Th 17 cells and then into plasma cells that release the AQP4-IgG autoantibody, as well as accelerate B cell development to plasmablasts [26]. We think that the primary cause of AQP4-related NMOSD is Interleukin 6 (IL-6), and that Th17 cell development stimulates IL-17 and IL-21. We also thought that the leakage of the BBB facilitates the migration of AQP4-IgG (binding to AQP4/complement cascade expression) from the periphery into the central nervous system. As we represented in Figure 1, As and OLG damage (demyelination) results from the activated complement and neutrophil/eosinophil infiltration. The before-mentioned GM metabolites production passes across the injured BBB membrane and regulates B and T lymphocytes, as represented in Figure 1. As we represented metabolites influence Treg and Th17 cell development and have a significant impact on T cells. Other authors in 2006 demonstrated that 3-oxoLCA, a derivative of Lithocholic Acid (LCA), inhibits Th17 cell differentiation by blocking the function of RORγt, whereas Th17 and Innate Lymphocyte Cell group 3 (ILC3) express RORγt selectively, resulting in autoimmune diseases and chronic inflammation [25,37].
Conclusion
However, by increasing the generation of OXPHOS and mROS and increasing the amount of Histone (H3K27) acetylation in the Foxp3 promoter, isoalloLCA, another derivative of LCA, causes Treg cell differentiation. This study’s primary finding is that the metabolites SCFAs, Trp, and BAs generated from GM are very significant in the immune cell metabolic network. The majority of researchers worldwide now acknowledge the role of GM in the pathophysiology of NMOSD. It can be used as a biomarker for the onset and progression of NMOSD and as a potential disease-modifying treatment, such as Fecal Microbiota Transplantation (FMT), to improve the prognosis of patients with NMOSD. To confirm or deny the benefit of FMT, we recommend conducting a clinical trial on individuals with NMOSD.
Declarations
Ethical considerations
This review was not considered ethical approval because all the data were extracted from previously published articles. Nevertheless, we obtained signed written consent from our patient for publishing her clinical information and images. Therefore, the Institutional Ethical Committee did not consider this study for additional ethical approval.
Declaration of anonymity
The authors certify that they did not reveal the names, surnames, or initials of any patient. Therefore, complete anonymity is guaranteed.
Availability of data and material
The data that support the findings of this study are available on reasonable request from the corresponding author.
Acknowledgement
We sincerely thank Dr Sibi Joseph for his enthusiastic support.
Competing Interest
The authors have not any conflict of interest to disclose. We declare no commercial or financial relationships construed as a potential conflict of interest.
Funding
The authors declared that they never received any financial support or personal collaboration that could have influenced the results reported in this paper.
References
- L. Bollo, C. Santoro, G. Libro, R. Pellicciari, D. Paolicelli, et al. Neuromyelitis optica spectrum disorders associated with systemic sclerosis: A case report and literature review, Neurol Sci, 43(2022):4015-4018.
- C.T. Tan, International consensus diagnostic criteria for neuromyelitis optica spectrum disorders, Neurol, 86(2016):491-492.
- C. Martin, T. Maurer, M.M. Mutizwa, Neuromyelitis optica with cutaneous findings: Case report and review of the literature, Dermatol, 230(2015):289-292.
- L. Misery, S. Genestet, F. Zagnoli, Neuromyelitis optica and skin, Dermatol, 238(2022):1-6.
- X. Chen, R. Fan, F. Peng, J. Liu, J. Huang, et al. Blood pressure and body fat percent in women with NMOSD, Brain Behav, 9(2019):01350.
- M.R. Ajmera, A. Boscoe, J. Mauskopf, S.D. Candrilli, Evaluation of comorbidities and health care resource use among patients with highly active neuromyelitis optica, J Neurol Sci, 384(2018):96-103.
- S. Paul, G.P. Mondal, R. Bhattacharyya, K.C. Ghosh, Neuromyelitis optica spectrum disorders, J Neurol, 420(2021):169-176.
- D.M. Wingerchuk, B. Banwell, J.L. Bennett, P. Cabre, W. Carroll, et al. International consensus diagnostic criteria for neuromyelitis optica spectrum disorders, Neurology, 85(2015):177-189.
- E. Carnero Contentti, J. Correale, Neuromyelitis optica spectrum disorders: From pathophysiology to therapeutic strategies, J Neuroinflammation, 18(2021):1-18.
- S.R. Hinson, S.F. Roemer, C.F. Lucchinetti, J.P. Fryer, T.J. Kryzer, Aquaporin-4-binding autoantibodies in patients with neuromyelitis optica impair glutamate transport by down-regulating EAAT2, J Exp Med, 206(2008):2473-2481.
- S.R. Hinson, S.J. Pittock, C.F. Lucchinetti, S.F. Roemer, J.P. Fryer, et al. Pathogenic potential of Igg binding to water channel extracellular domain in neuromyelitis optica, Neurol, 69(2007):2221-2231.
- Y. Takeshita, B. Obermeier, A.C. Cotleur, Effects of neuromyelitis optica-Igg at the blood-brain barrier in vitro, Neurol Neuroimmunol Neuroinflammation, 4(2016):311.
- I. Kleiter, A. Gahlen, N. Borisow, K. Fischer, K.D. Wernecke, et al. Neuromyelitis optica: Evaluation of 871 attacks and 1,153 treatment courses, Ann Neurol, 79(2016):206-216.
- B.G. Weinshenker, What is the optimal sequence of rescue treatments for attacks of neuromyelitis optica spectrum disorder? Ann Neurol, 79(2016):204-205.
- D.M. Wingerchuk, Neuromyelitis optica, Int MS J, 13(2006):42-50.
- S. Joseph, L.D.F. Ibanez-Valdes, H. Foyaca-Sibat, Neuromyelitis optica spectrum disorder associated with arterial hypertension and pityriasis versicolor. A novel presentation, Clin Schizophr Relat Psychoses, (2022).
[Crossref]
- Abbadessa, Gianmarco, G. Miele, E. Maida, G. Minervini, et al. Optimal retreatment of rituximab for neuromyelitis optica spectrum disorder: A systematic review, Mult Scler Relat Dis, 63(2022):103926.
- J. Ma, H. Yu, H. Wang, X. Zhang, K. Feng, Evaluation of the effect of empirical attack-preventive immunotherapies in neuromyelitis optica spectrum disorders: An updated systematic review and meta-analysis, J Neuroimmunol, 363(2022): 577790.
- J. Palace, D.M. Wingerchuk, K. Fujihara, A. Berthele, C. Oreja-Guevara, et al. Benefits of eculizumab in Aqp4+ neuromyelitis optica spectrum disorder: Subgroup analyses of the randomized controlled phase 3 prevent trial, Mult Scler Relat Disord, 47(2021):102641.
- J. Palace, I. Leite, A. Jacob, A practical guide to the treatment of neuromyelitis optica, Pract Neurol, 12(2012):209-214.
- S.R. Hinson, S.J. Pittock, C.F. Lucchinetti, S.F. Roemer, J.P. Fryer, et al. Pathogenic potential of igg binding to water channel extracellular domain in neuromyelitis optica, Neurol, 69(2007):2221-2231.
- F.S. Humberto, Bilateral putaminal haemorrhage and blindness in times of the coronavirus pandemic and dysbiosis: Case report and literature review, Clin Schizophr Relat Psychoses, 15S(2021).
- L.F.I. Valdes, H.F. Sibat, Comorbidity of alcohol use disorder and neurocysticercosis aggravated by dysbiosis, J Drug Alc Res, 13(2024).
- S.Q. Yao, X. Yang, L.P. Cen, S. Tan, The role of gut microbiota in neuromyelitis optica spectrum disorder, Int J Mol Sci, 25(2024):3179.
- L.F.I. Valdes, H.F. Sibat, Novel hypotheses on the role of oligodendrocytes in neurocysticercosis and their implications for drug development: A comprehensive review, J Drug Alc Res, 13(2024).
- M. Varrin-Doyer, C.M. Spencer, U. Schulze-Topphoff, P.A. Nelson, R.M. Stroud, et al. Aquaporin 4-specific T cells in neuromyelitis optica exhibit a Th17 bias and recognize clostridium ABC transporter, Ann Neurol, 72(2012):53-64.
- L. Pandit, L.M. Cox, C. Malli, A. D'Cunha, T. Rooney, et al. Clostridium bolteae is elevated in neuromyelitis optica spectrum disorder in India and shares sequence similarity with AQP4, Neurol Neuroimmunol Neuroinflammation, 8(2021):e907.
- S.S. Zamvil, C.M. Spencer, S.E. Baranzini, B.A.C. Cree, The gut microbiome in neuromyelitis optica, Neurotherapeutics, 15(2018):92-101.
- S. Markovic-Plese, B. Hemmer, Y. Zhao, R. Simon, C. Pinilla, et al. High level of cross-reactivity in influenza virus hemagglutinin-specific CD4+ T-cell response: Implications for the initiation of autoimmune response in multiple sclerosis, J Neuroimmunol, 169(2005):31-38.
- T. Parks, L. Barrett, N. Jones, Invasive streptococcal disease: A review for clinicians, Br Med Bull, 115(2015):77-89.
- S. Landwehr-Kenzel, P. Henneke, Interaction of Streptococcus agalactiae and cellular innate immunity in colonization and disease, Front Immunol, 5(2014):519.
- J. Gong, W. Qiu, Q. Zeng, X. Liu, X. Sun, et al. Lack of short-chain fatty acids and overgrowth of opportunistic pathogens define dysbiosis of neuromyelitis optica spectrum disorders: A Chinese pilot study, Mult Scler, 25(2019):1316-1325.
- C. Cui, S. Tan, L. Tao, J. Gong, Y. Chang, et al. Intestinal barrier breakdown and mucosal microbiota disturbance in neuromyelitis optical spectrum disorders, Front Immunol, 11(2020):2101.
- Z. Shi, Y. Qiu, J. Wang, Y. Fang, Y. Zhang, et al. Dysbiosis of gut microbiota in patients with neuromyelitis optica spectrum disorders: A cross-sectional study, J Neuroimmunol, 339(2020):577126.
- J. Zhang, Y.F. Xu, L. Wu, H.F. Li, Z.Y. Wu, Characteristic of gut microbiota in southeastern Chinese patients with neuromyelitis optica spectrum disorders, Mult Scler Relat Disord, 44(2020):102217.
- C. Ding, Y. Hong, Y. Che, T. He, Y. Wang, et al. Bile acid restrained T cell activation explains cholestasis aggravated hepatitis B virus infection, FASEB J, 36(2022):e22468.
- I.I. Ivanov, B.S. McKenzie, L. Zhou, C.E. Tadokoro, A. Lepelley, et al. The orphan nuclear receptor RORgammat directs the differentiation program of proinflammatory IL-17+ T helper cells, Cell, 126(2006):1121-1133.
Copyright: © 2024 Lourdes de Fatima Ibanez Valdes, et al. This is an open access article distributed under the terms of the Creative Commons Attribution License, which permits unrestricted use, distribution, and reproduction in any medium, provided the original work is properly cited.