Research Article - Journal of Drug and Alcohol Research ( 2022) Volume 11, Issue 5
In-Vivo Antinociceptive Activity and In-Silico Molecular Docking of Selected Phytoconstituents of Methanolic Extract of Hypericum Japonicum
Shaik Aminabee*, Raveesha Peeriga, V. Adithya, M. Mohansai, Shaherbanu, K. Harshitha, K. Himaja Kasthuri, M. Lakshmi Priya, G. Chandini Naga Mallika and Lakshmana Rao AtmakuriShaik Aminabee, Department of Pharmacology, V. V. Institute of Pharmaceutical Sciences, India, Email: aminaammi786@gmail.com
Received: 02-May-2022, Manuscript No. jdar-22-63494; Editor assigned: 04-May-2022, Pre QC No. jdar-22-63494 (PQ); Reviewed: 18-May-2022, QC No. jdar-22-63494; Revised: 23-May-2022, Manuscript No. jdar-22-63494 (R); Published: 30-May-2022, DOI: 10.4303/jdar/236178
Abstract
This research work was carried out to analyse and evaluate the antinociceptive activity of methanolic extract of Hypericum Japonicum (MEHJ) and in-silico molecular docking of selected phytoconstitutents with cyclooxygenase- 2 (COX-2) enzyme along with absorption (A), distribution (D), metabolism (M), excretion (E) and toxicity (T) studies. In-vivo antinociceptive activity was performed by hot plate method, tail immersion method and acetic acid induced writhing response method in rat. In-silico molecular docking was done by using Autodock Vina and Discovery Studio Visualizer. Absorption, distribution, metabolism, excretion and toxicity (ADMET) studies were examined by Swiss ADME software. The results proved that methanolic extract of Hypericum Japonicum has dose dependent antinoceptive activity at all doses. Among all the phytoconstitutents saroaspidin B has very best docking rate of -7.1 kcal/mol which was better virtually than standard celecoxib which has docking rate of -7.4 kcal/mol. This shows that there is good binding affinity between ligand and receptor than the standard i.e celecoxib. ADMET evaluation using swissADME and admeSAR software assures that saroaspidin B has followed all the 5 Lipinski’s guidelines suggesting that it is safety for consumption. Hence by this research, we conclude that Hypericum Japonicum can be a potent agent as antinociceptive activity and further studies are required to for the development of performance of saroaspidin B.
Keywords
Hypericum Japonicum; Kielcorin; Mesuaxanthone B; Analgesic; Celecoxib
Abbrevations
(TAE) Tannic Acid Equivalent; (GAE) Gallic Acid Equivalents; (CE) Catechin Equivalents; (AE) Atropine Equivalent; (RT) Retention Time; (PA) Peak Area
Introduction
To recognize the location that is damaged and harmed by numerous stimulations, pain is a tool that is beneficial in body’s immune system. For the treatment of pain, many drugs like non-steroidal anti-inflammatory drugs (NSAIDs), analgesics opioid in nature, opioid anaesthetics and steroidal medicines are used [1]. They are having many harmful effects like kidney failure, liver damage, cardiac problems, high blood pressure, erectile dysfunction, skin degeneration, manic depression, reduced bone density, constipation, abscess and respiratory problems. So it gained importance for herbal based antinoceptive drug which can be available at low cost, more potent and has less negative effects [2]. In order to generate highly active compound with minimum adverse effects, drug design has become a vital tool in medicinal chemistry field where novel compounds are synthesized by chemical or molecular modification of lead moiety. In-silico docking method was a huge breakthrough in drug design and development to predict therapeutic efficacy of the novel molecules [3]. To design new drugs, molecular docking has become an essential element where selected protein will show binding affinity for ligand. To understand chemical properties and drug receptor interactions, In-silico docking methods are largely useful [4]. Hypericum Japonicum is an annual herb flowering plant belonging to the family hypericaceae. It is only 2-5 cm long. Its stems are green, 4 angled and 2-52 mm long internodes that exceed the leaves. The leaves are persistent, spreading and sessile. This species is 30 flowered and flowers are branched upto 3 nodes. The flowers are 4-8 mm in diameter and petals are bright orange or yellow. The stamens are 5-30 in number arranged in irregular groups. Seeds are 50 mm long approximately. It is distributed in India, China, Laos, Japan, Vietnam, Myanmar, Thailand, Malaysia, and Indonesia, Philippines to New Guinea, New Zealand, and Australia. It is well grown in good drained and soil that retains moisture. Flourish and semi shade under sun. Sunny region is better foe flowers. Mostly this species was distributed in wet soils. The plant is harvested from the wild for local use as a medicine. The plant is antiphlogistic, alternative, astringent, febrifuge, depurative, vulnerary and stomachic. For use it can be boiled with water. Also used in the therapy of dysentery, appendicitis, acute hepatitis, asthma, pain in the liver region, abscesses and boils. Also used as a styptic. In the treatment of malaria it was combined with Zingiber officinale (ginger) and ash salt. It is also having antitumour activity. Applied externally, it is used to treat leech, wounds, swellings, snake bites, scrofula abscesses and fungal skin diseases.
Materials and Methods
Collection and identification of plant material
Whole plant of Hypericum Japonicum was collected from Tirumala hills, Tirupathi, Andhra Pradesh. The plant was confirmed and acknowledged by Prof. K. Madhava Chetty, Plant Taxonomist, Professor, Department of Botany, SV University, Tirupathi, and Andhra Pradesh, India [5]. Hypericum Japonicum was thoroughly washed and cleaned with water, air dry under room temperature and grounded into powdered using home appliance. It was preserved for pharmacognostical and pharmacological study.
Preparation of extracts
By using home appliances the shade dried Hypericum Japonicum was powdered. Soxhlet apparatus was used for extraction of coarse powder (1000 gm) with methanol. The final extract was filtered. In rotating evaporator the extract was concentrated by vaporization of solvent and maintained in refrigerator [6].
Phytochemical analysis
For the existence of saponins, alkaloids, terpenoids, glycosides, flavonoids, tannins, carbohydrates, proteins, amino acids and fixed oils phytochemical analysis of extracts were carried out by different methods [7].
Experimental animals
From livestock building of Vallabhaneni Venkatadri Institute of Pharmaceutical Sciences, Gudlavalleru albino wistar rats (180-200 gm) are obtained. Animals are fasted for a period of 12 hrs before and after treatment water was ad libitum.
Acute toxicity studies
As per OECD guidelines 423, acute toxic studies are performed on MEHJ taking albino mice of body weight 20-25 gm as experimental animals. Adult mice which are healthy are divided into groups and each group contained 5 mice. Each group received MEHJ at different doses of 5, 50, 200 and 300 mg/kg body weight orally. For mortality and physical/ behavioural changes animals were observed for over 14 days [8].
Evaluation of antinociceptive activity
Acetic acid induced writhing response: The method described by Dharmasiri et al., was followed for the evaluation of analgesic activity of MEHJ with slight modifications. Number of rats in each group are 5. Normal saline (2 ml/kg) was given to control group orally. Standard group received diclofenac 5 mg/kg and test groups received MEHJ at different doses of 50, 100, 200, 400 mg/kg. After 30 min, all the animals in different groups received 0.7% 10 ml/kg acetic acid solution intraperitoneally [9]. The writhing’s induced by acetic acid include back limb stretching’s and stomach restrictions which are considered for about 30 min with 5 min latency period. A notable deduction of writhes in test group animals analogized to normal saline group (control) was appraised as a significant antinociceptic reaction.
Eddy’s hot plate test
Six groups of animals are taken, each group contain 5 animals. Control is group I. Standard is group II and received tramadol 20 mg/kg intraperitoneally. Test groups are III, IV, V and VI and they received MEHJ orally of doses 50, 100, 200 and 400 mg/kg body weight respectively. All the animals 1 hour after their respective treatments are positioned separately on the hot plate kept at 55°C. The response of the animal to pain stimulus either by jumping or paw licking (whichever appeared first) was noted as the time at which the animal reacted. 15 seconds was the cut off time for the reaction [10]. The response was noted before (0 min) and after drug administration for 0.5, 1, 1.5, 2 and 3 hr.
Tail immersion test
Six groups of animals are taken, each group contain 5 animals. Control is group I. Standard is group II and received tramadol 20 mg/kg intraperitoneally. Test groups are III, IV, V and VI and they received MEHJ orally of doses 50, 100, 200 and 400 mg/kg body weight respectively. After 1 hour of therapy, the tip of animal tail approximately up to 5 cm was dipped in hot water which was sustained at 58°C. Unexpected removal of tail from 58°C hot water was noted as the response time. In order to circumvent injury to the tail cut off time was 10 seconds for all groups of animals [11]. To assess response to noxious stimulus, the time taken for flicking the tail was noted.
In-silico molecular docking
Computational study: The molecular docking studies are carried on cyclooxygenase-2 (COX-2) enzyme. The structure of COX-2 was acquired via protein data bank (ID: 4PH9). Three dimensional structure of COX-2 (4PH9) was given in Figure 1. The plant constituents from Hypericum japonicum was collected from literature wok testimonial [12].
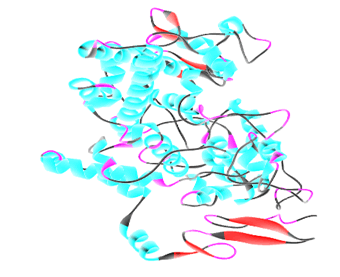
Figure 1: Three dimensional structure of COX-2 (4PH9)
Preparation of ligand structures
Selected ligand structures are downloaded from PubChem database in 3D SDF format. 3D SDF chemical structures of ligands are transformed to .pdb format by using BIOVIA Discovery Studio Visualizer. After that ligand in .pdb format is opened in AutoDock Tools (ADT) for amplification Gasteiger moderation and rotatable bonds [13]. Ligand structure in .pdb format is converted to .pdbqt format by using AutoDock vina. Three dimensional structures of selected ligands are given in Figure 2.
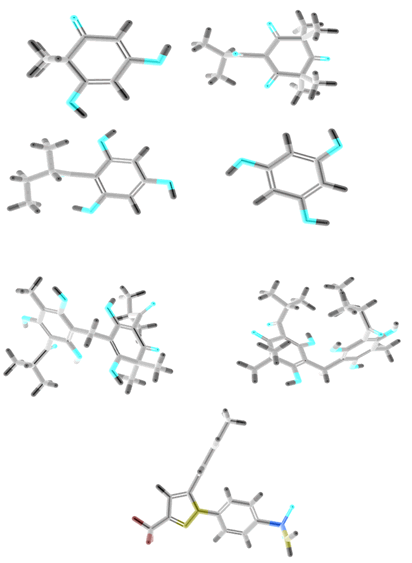
Figure 2: Three dimensional structure of ligands A. Filicinic acid, B. Flavesone, C. Multifidol, D. Phloroglucinol, E. Saroaspidin A, F. Saroaspidin B, G. Celecoxib.
Preparation of COX-2 protein
The 3D structure of protein COX-2 (Id: 4PH9) was downloaded from RCSB PDB (Research Collaboratory for Structural Bioinformatics Protein Data Base). The COX-2 was opened in BIOVIA Discovery Studio Visualizer. Then remove water molecules, next find the list of ligands inside by define and edit the binding sites. Binding sphere is generated; make sure that all the ligands are enclosed in the binding sphere. Click on the sphere, it turns yellow in colour and then right click it to get the attributes x, y and z. Note x, y and z parameters in the notepad [14]. Then remove the ligand and add polar hydrogen molecules. Now this is the prepared protein so save this as protein. pdb format. Open protein.pdb in Auto Dock vina, go to grid macromolecule choose. Select protein molecule. Initializing pop up will appear on screen. Now save this as protein.pdbqt.
Docking methodology
Ligand.pdbqt and protein.pdbqt are ready now. In command prompt, by using various command instructions complex formed is split into poses. Protein.pdbqt and poses are opened in BIOVIA Discovery Studio Visualizer. By using receptor-ligand interaction we can find out which pose is having best binding affinity with receptor [15]. The rest of the poses are deleted. 2D and 3D structures of the selected pose are taken and results are interpreted.
ADMET analysis
Pharmacokinetic properties of the ligands in order to understand the function of ligands inside the body ADMET analysis was done by using admetSAR. Lipinski’s rule of five (RO5), is a rule that a chemical compound with certain physical properties, chemical properties and pharmacological activity that can be orally active drug in humans to evaluate drug likeness [16].
The rule outlines the pharmacokinetic of drugs such as absorption (A), distribution (D), metabolism (M) and excretion (E) in the human body but pharmacodynamics properties of a drug cannot be confirmed. In our study, we used swissADME and admeSAR software to assess the ADME and noxious effects of the isolated compounds from Hypericum japonicum. In this swissADME and admeSAR software, we used the canonical smiles to for ADME/T analysis.
Statistical analysis
By using one way ANOVA and multiple comparisons by Dunnetts test data comparison was done as mean ± SEM. At P<0.05, <0.01 and <0.001 values are considered to be significant statistically.
Results
Phytochemical analysis
The phytochemical screening of methanolic extract of Hypericum Japonicum revealed the appearance of saponins, alkaloids, terpenoids, glycosides, flavonoids, tannins, carbohydrates, proteins, amino acids and fixed oils (Table 1). Specific phytoconstituents present in Hypericum Japonicum are obtained from literature survey [17].
Table 1: Phytochemical screening of successive solvent extraction of MEHJ.
Phytoconstituents | Method | Methanolic extract |
---|---|---|
Flavonoids | Shinoda Test | + |
Zn. Hydrocholride Test | + | |
Lead acetate Test | + | |
Alkaloids | Wagner Test | + |
Hager’s Test | + | |
Steroids | Salkowski Test | + |
Saponins | Foaming Test | + |
Tannins & Phenols | Fecl3 Test | + |
Pot.Dichromate Test | + | |
Volatile Oil | Stain Test | + |
Glycoside | Keller-Killani Test | + |
Carbohydrates | Molish Test | + |
Acid Compounds | Litmus Test | - |
Proteins | Biuret | + |
Amino Acids | Ninhydrin Test | + |
In-vivo analgesic activity
In acetic acid induced writhing response, all rats in different groups displayed writhings in significant number compared to that of control. Standard group treated with diclofenac showed low number of writhings collated to other treated groups. Between the treated groups, group treated with MEHJ 400 mg/kg showed significant inhibition of writhings. The results are presented in Table 2.
Table 2: Effect of methanolic extract of Hypericum Japonicum by acetic acid induced writhing response.
Experimental Groups | Dose (mg/kg) | Number of Writhes | % inhibition |
---|---|---|---|
Control (acetic acid) | 0 | 25.2 ± 2.11 | 0 |
Diclofenac | 5 | 7.63 ± 1.56 | 69.72 |
MEHJ | 50 | 23.12 ± 1.36 | 8.2 |
MEHJ | 100 | 19.25 ± 0 .99 | 23.61 |
MEHJ | 200 | 18.36 ± 1.11 | 27.14 |
MEHJ | 400 | 10.11 ± 1.45 | 59.88 |
Results of antinociceptive activity by Eddys hot plate method are presented in Table 3. Different doses of MEHJ extract and standard group showed notable elevation in latency time collated to control group at 1.5 hr. between the extracts MEHJ (400 mg/kg) manifested the best elevation in latency time.
Table 3: Effect of methanolic extract of Hypericum Japonicum by hot plate method.
Treatment | Reaction time | |||||
---|---|---|---|---|---|---|
0 min | 0.5 hr | 1 hr | 1.5 hr | 2 hr | 3 hr | |
Normal Saline (2 ml/kg) | 3.17 ± 0.01 | 3.12 ± 0.05 | 3.18 ± 0.08 | 3.20 ± 0.04 | 3.21 ± 0.08 | 3.23 ± 0.05 |
Tramodol (20 mg/kg) | 3.16 ± 0.05 | 5.12 ± 0.12 (62.02) | 5.53 ± 0.05 (75.00) | 5.82 ± 0.09 (84.17) | 4.98 ± 0.11 (57.59) | 4.85 ± 0.08 (53.48) |
MEHJ (50 mg/kg) | 3.34 ± 0.06 | 3.41 ± 0.08 (2.09) | 3.62 ± 0.07 (8.38) | 3.99 ± 0.08 (19.46) | 3.68 ± 0.07 (10.17) | 3.55 ± 0.12 (6.28) |
MEHJ (100 mg/kg) | 3.21 ± 0.11 | 3.32 ± 0.09 (3.42) | 4.23 ± 0.05 (31.77) | 4.45 ± 0.11 (38.62) | 4.26 ± 0.12 (32.71) | 3.91 ± 0.11 (21.8) |
MEHJ (200 mg/kg | 3.43 ± 0.06 | 3.81 ± 0.08 (11.07) | 4.13 ± 0.07 (20.4) | 4.82 ± 0.09 (40.52) | 4.29 ± 0.14 (25.07) | 4.09 ± 0.12 (19.24) |
MEHJ (400 mg/kg | 3.53 ± 0.07 | 4.23 ± 0.09 (19.83) | 4.84 ± 0.08 (37.11) | 5.75 ± 0.06 (62.88) | 5.23 ± 0.15 (48.15) | 4.96 ± 0.11 (40.5) |
In Table 4, the results of analgesic activity by tail immersion test are represented. Different doses of MEHJ extract and standard group showed notable elevation in latency time collated to control group at 1.5 hr. Between the extracts MEHJ (400 mg/kg) manifested the best elevation in latency time.
Table 4: Effect of methanolic extract of Hypericum Japonicum by tail immersion test.
Treatment | Reaction time | |||||
---|---|---|---|---|---|---|
0 min | 0.5 hr | 1 hr | 1.5 hr | 2 hr | 3 hr | |
Normal Saline (2 ml/kg) | 2.51 ± 0.08 | 2.62 ± 0.07 | 2.70 ± 0.07 | 2.53 ± 0.04 | 2.49 ± 0.11 | 2.56 ± 0.12 |
Tramodol (20 mg/kg) | 2.39 ± 0.11 | 3.85 ± 0.12 (61.08) | 4.36 ± 0.09 (82.42) | 4.21 ± 0.12 (76.15) | 3.99 ± 0.04 (66.94) | 3.76 ± 0.14 (57.32) |
MEHJ (50 mg/kg) | 2.36 ± 0.09 | 2.45 ± 0.07 (3.81) | 2.54 ± 0.09 (7.62) | 2.61 ± 0.14 (10.59) | 2.51 ± 0.09 (6.35) | 2.45 ± 0.15 (3.81) |
MEHJ (100 mg/kg) | 2.33 ± 0.07 | 2.49 ± 0.09 (6.86) | 2.65 ± 0.08 (13.73) | 2.71 ± 0.09 (16.3) | 2.58 ± 0.13 (10.72) | 2.45 ± 0.09 (5.15) |
MEHJ (200 mg/kg | 2.35 ± 0.06 | 2.85 ± 0.11 (21.27) | 3.11 ± 0.07 (32.34) | 3.48 ± 0.11 (48.08) | 3.23 ± 0.07 (37.44) | 2.89 ± 0.08 (22.97) |
MEHJ (400 mg/kg | 2.29 ± 0.13 | 3.32 ± 0.13 (44.97) | 3.62 ± 0.06 (58.07) | 3.74 ± 0.03 (63.31) | 3.59 ± 0.09 (56.76) | 3.21 ± 0.06 (40.17) |
In-silico study for the evaluation of analgesic activity
Tables 5 and 6 represents the physicochemical properties of phytoconstitutents of Hypericum Japonicum required for drug likeliness and ADME/T analysis. By analyzing the results given in these tables we can predict the eligibility of a plant constituent to pass as a safe compound.
Table 5: Drug likeliness analysis of separated phytoconstituents from Hypericum Japonicum.
Compound | Total Mol. Weight | H-Acceptors | H-Donors | cLogP | Rotatable Bonds | Molar Refractivity | No. of Deviations |
---|---|---|---|---|---|---|---|
1,2,5-Trihydroxyxanthone | 244.2 | 5 | 3 | 1.99 | 0 | 66.06 | 0 |
Euxanthone | 228.2 | 4 | 2 | 2 | 0 | 64.04 | 0 |
Filicinic acid | 154.16 | 3 | 2 | 1.02 | 0 | 40.59 | 0 |
Flavesone | 252.31 | 4 | 0 | 2.3 | 2 | 67.58 | 0 |
Isoquercitin | 464.38 | 12 | 8 | 2.11 | 4 | 110.16 | 2 |
Kielcorin | 436.41 | 8 | 2 | 3.45 | 4 | 116.32 | 0 |
Mesuaxanthone B | 244.2 | 5 | 3 | 1.43 | 0 | 66.06 | 0 |
Multifidol | 210.23 | 4 | 3 | 1.55 | 3 | 57.13 | 0 |
Phloroglucinol | 126.11 | 3 | 3 | 0.66 | 0 | 32.51 | 0 |
Saroaspidin A | 446.49 | 8 | 5 | 2.52 | 6 | 120.16 | 0 |
Saroaspidin B | 460.52 | 8 | 5 | 2.65 | 7 | 124.96 | 0 |
Sarothralin G | 602.71 | 8 | 5 | 4.89 | 11 | 172.54 | 1 |
Table 6: ADMET properties of separated phytoconstituents from Hypericum Japonicum.
Compound | HIA | BBB | AMES Toxicity | Carcinogenicity | LD50 in rats |
---|---|---|---|---|---|
Filicinic acid | 0.9831 | 0.918 | Non-toxic | Non-carcinogenicity | 2.538 |
Flavesone | 0.9825 | 0.984 | Non-toxic | Non-carcinogenicity | 1.455 |
Multifidol | 0.996 | 0.3391 | Non-toxic | Non-carcinogenicity | 5.282 |
Phloroglucinol | 0.9853 | 0.7298 | Non-toxic | Non-carcinogenicity | 4.374 |
Saroaspidin A | 0.9916 | 0.2444 | Non-toxic | Non-carcinogenicity | 5.281 |
Saroaspidin B | 0.9916 | 0.8103 | Non-toxic | Non-carcinogenicity | 5.846 |
Docking rates are given in Table 7. Saroaspidin B had the most excellent docking score of -7.1 kcal/mol, which showed both hydrogen bond and hydrophobic interactions with COX-2 enzyme. The standard celecoxib revealed the docking score of -7.4 kcal/mol which is comparable to saroaspidin B.
Table 7: Binding affinities of separated phytoconstituents from Hypericum Japonicum.
Compound | 1 | 2 | 3 | 4 | 5 | 6 | 7 | 8 | 9 |
---|---|---|---|---|---|---|---|---|---|
1,2,5-Trihydroxyxanthone | -8.2 | -7.8 | -7.3 | -7.1 | -7 | -7 | -6.6 | -6.5 | -6.4 |
Euxanthone | -7.7 | -7.7 | -7.1 | -6.6 | -6.5 | -6.4 | -6.4 | -6.4 | -6.4 |
Filicinic acid | -6.3 | -6.3 | -6.1 | -6.1 | -5.7 | -5.7 | -5.4 | -5.3 | -5.3 |
Flavesone | -6.3 | -5.5 | -5.4 | -5.4 | -5 | -4.9 | -4.9 | -4.9 | -4.5 |
Isoquercitin | -7.8 | -7.8 | -7.7 | -7.7 | -7.7 | -7.6 | -7.5 | -7.4 | -7.2 |
Kielcorin | -8.2 | -8 | -7.9 | -7.8 | -7.6 | -7.1 | -6.9 | -6.7 | -6.6 |
Mesuaxanthone B | -8.2 | -7.4 | -6.7 | -6.7 | -6.4 | -6.3 | -6.3 | -6.3 | -6.2 |
Multifidol | -6.2 | -6.2 | -6.2 | -6 | -6 | -5.7 | -5.7 | -5.7 | -5.7 |
Phloroglucinol | -5.3 | -5.3 | -5.3 | -5.3 | -5.3 | -5.3 | -5.3 | -5.3 | -5 |
Saroaspidin A | -6.8 | -6.5 | -6.4 | -6.4 | -6.4 | -6.3 | -6.3 | -6.3 | -5.9 |
Saroaspidin B | -7.1 | -7 | -6.9 | -6.7 | -6.7 | -6.6 | -6.6 | -6.6 | -6.6 |
Sarothralin G | -8.9 | -8.4 | -8.4 | -8.3 | -8.3 | -8.1 | -8 | -7.9 | -7.9 |
Celecoxib | -7.4 | -7.0 | -6.9 | -6.6 | -6.5 | -6.3 | -6.2 | -6.1 | -6.1 |
Protein ligand interactions revealing hydrogen binding and hydrophobic interactions are given in Table 8 and published in Figures 3 and 4.
Table 8: Interactions of COX-2 with ligands at receptor sites.
Compound | Hydrogen Binding Interaction | Hydrophobic Interaction |
---|---|---|
Filicinic acid | TYR (A:386), MET (A:523) | --- |
Flavesone | THR (A:213), HIS (A:387) | --- |
Multifidol | TRP (A:388) | LEU (A:392), ALA (A:200) |
Phloroglucinol | TRP (A:388), ALA (A:203), HIS (A:389), HIS (A:208) | --- |
Saroaspidin A | HIS (A:387) | HIS (A:215), VAL (A:292), ARG (A:223) |
Saroaspidin B | HIS (A:208), ASN (A:383), GLN (A:455) | THR (A:213), HIS (A:387) |
Celecoxib | GLN (A:290), ASN (A:383), THR (A:213) | VAL (A:448) |
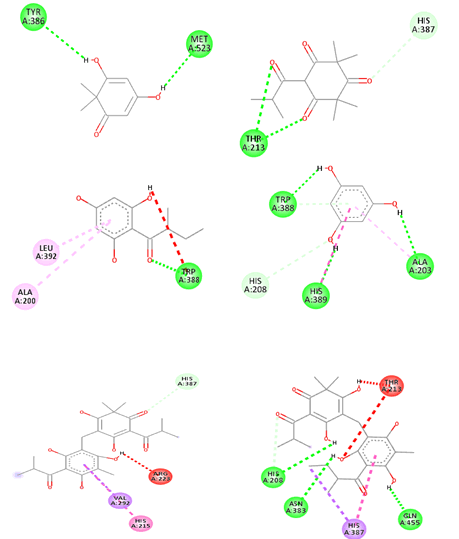
Figure 3: Two dimensional interactions of ligands with COX-2 (4PH9) A. Filicinic acid, B. Flavesone, C. Multifidol, D. Phloroglucinol, E. Saroaspidin A, F. Saroaspidin B G. Celecoxib.
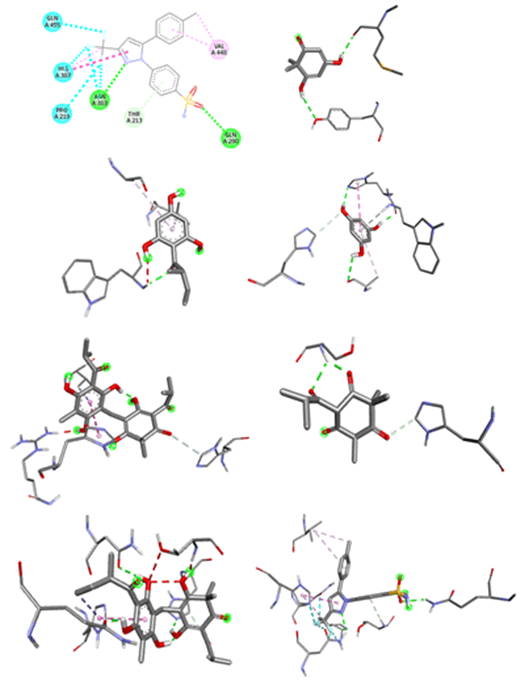
Figure 4: Three dimensional interactions of ligands with COX-2 (4PH9) A. Filicinic acid, B. Flavesone, C. Multifidol, D. Phloroglucinol, E. Saroaspidin A, F. Saroaspidin B, G. Celecoxib.
Discussion
By extraction of different plant parts various phytoconstituents are obtained that are responsible for therapeutic activity when used in medical purpose. For pharmacological action secondary metabolites are vitally responsible. Practically all the plants primary metabolites are established in every plant not all the secondary metabolites were found, they differ from plant to plant. So this is the reason that all plants do not show every kind of therapeutic or pharmacological reaction. Hence identification of phytoconstituent is the major step in the area of drug discovery. In the evaluation of in-vivo analgesic activity by acetic acid induced writhing response, from tissue phospholipids there is liberation of arachidonic acid (AA) which is considered a pain stimuli when 0.7% acetic acid is injected intra peritoneally. It is established that prostaglandins (PG) pathway or acid sensing ion channels or peritoneal most cells are responsible for induction of peripheral pain [18]. Peripheral analgesic effect of MEHF is given in Table 2. Opioid receptor agonist and centrally acting analgesics are effectively evaluated by Hot plate method and Tail immersion test. It is know that spinal and supra spinal receptors are the targets for opioid receptor agonist. For thermal induced nociception μ receptor agonist are more effective among these opioid receptor agonists. Hence this is the reason why hot plate method and tail immersion test are appraised to be very effective in the evaluation of peripheral analgesic activity [19]. Antinociceptive activity of MEHJ by hot plate method and tail immersion test are represented in Tables 3 and 4 respectively.
To identify the potential phytoconstituents from MEHJ for antinociceptive activity, first we investigated the compatibility of a phytoconstituent to be used as drug molecule. For this objective, some parameters are taken into consideration like molecular weight (<500 gm/ml), hydrogen bond acceptor (≤10), hydrogen bond donor (≤5), clogP (<5) and molar refractivity (40-130) [20]. These values are given in Tables 5 and 6. 6 out of 12 compounds have the capability to be used as a drug molecule. Next, we illustrated in-silico molecular docking rate, glide energy rate and glide e-model score on phytoconstituents of MEHJ with COX-2 enzyme in order to evaluate antinociceptive activity of Hypericum Japonicum in molecular level [21]. Selective COX-2 inhibitors are preferable option to stop pain stimulus as COX- 2 enzyme is the main culprit for acute pain [22]. Since COX-2 inhibitors are having extensive scope of adverse effects, finding replacement for selective COX-2 inhibitors is obligatory [23]. In our research saroaspidin B manifested very appreciable docking scale of -7.1 kcal/mol against COX-2 enzyme where as our standard drug celecoxib manifested -7.4 kcal/mol docking score which can be compared to our phytoconstituents. So, it may be possible that these phytoconstitutents saroaspidin B behave as favourable hit molecules for selective COX-2 inhibitors [24].
Conclusion
Medical plant had extensive scope of activity so they are considered as best source of medications for humans. It is our interest to select the plant, isolate the suitable phytoconstituents for the desirable activity, enhance them and formulate that phytoconstituent better with good potency and lesser side effects. In this study, we found that methanolic extract of Hypericum Japonicum has significant in-vivo antinociceptive activity. In addition to that, in-silico molecular docking and ADMET analysis were performed on various phytoconstituents present in MEHJ to find out the suitable phytoconstituent responsible for this antinociceptic activity. Our investigations revealed that saroaspidin B had good possibility to act as selective COX-2 inhibitor. In order to establish these phytoconstitutents as potent antinoceptive drug molecules vigorous study is suggested as this is a crude research.
Acknowledgements
Authors are sincerely thankful to Dr. K. Madhava Chetty, Plant Taxonomist, Department of Botany, SV University, Tirupati, India for authentification of plant materials. Authors are thankful to Vallabhaneni Venkatadri Institute of Pharmaceutical Sciences, Gudlavalleru, India for providing necessary facilities of research work.
Conflict of Interest
None.
References
- L. Boursinos, T. Karachalios, L. Poultsides, K. Malizos, Do steroids, conventional non-steroidal anti-inflammatory drugs, and selective Cox-2 inhibitors adversely affect fracture healing, J Musculoskelet Neuronal Interact, 9 (2009), 44-52.
- S. C. Koech, R. O. Ouko, N. M. Michael, M. M. Ireri, M.P. Ngugi, et al., Analgesic activity of dichloromethanolic root extract of Clutia abyssinica in swiss albino mice, Nat Prod Chem Res, 5 (2017) 2.
[Crossref] [Google Scholar] [PubMed]
- R. A. Friesner, J. L. Banks, R. B. Murphy, T. A. Halgren, J. J. Klicic, et al., Glide: A new approach for rapid, accurate docking and scoring. Method and assessment of docking accuracy, J Med Chem ACS Publications, 47 (2004) 1739–49.
[Crossref] [Google Scholar] [PubMed]
- P. Singh, F. Bast, In silico molecular docking study of natural compounds on wild and mutated epidermal growth factor receptor, Med Chem Res, 23 (2014) 5074-85.
[Crossref] [Google Scholar] [PubMed]
- K. Madhava Chetty, K. Sivaji, K. Tulasi Rao, Flowering plants of Chittoor District, Andhra Pradesh, India 4th edition, Student offset Printers, Tirupathi, (2013). [Crossref]
[Google Scholar] [PubMed]
- S. Devi, a study on high valued medicinal plants of Tirumala Hills, (2011).
[Crossref] [Google Scholar] [PubMed]
- K. R. Khandelwal, Practical pharmacognosy techniques and experiments, (2000) 149-156. [Crossref]
- E. Walum, Acute oral toxicity, Environ Health Perspect, 106 (1998) 497-503.
[Crossref] [Google Scholar] [PubMed]
- R. Koster, M. Anderson, E. J. De-Beer, Acetic acid analgesic screening, Fed Proc, 18 (1959) 412-417.
[Crossref] [Google Scholar] [PubMed]
- W. Toma, J. S. Graciosa, C. A. Hiruma-Lima, F. D. P. Andrade, W. Vilegas, et al., Evaluation of analgesic and antiedematogenic activities of Quassia Amara bark extract, J Ethnopharmacol, 85 (2003) 19-23.
[Crossref] [Google Scholar] [PubMed]
- Y. Chen, S. Tao, F. Zeng, L. Xie, Z. Shen, Antinociceptive and anti-inflammatory activities of Schefflera octophylla extracts, J Ethnopharmacol. 17 (2015) 42-50.
- O. Trott, A. J. Olson, AutoDock Vina: Improving the speed and accuracy of docking with a new scoring function, efficient optimization, and multithreading, J Comput Chem, 31 (2010) 455-461.
[Crossref] [Google Scholar] [PubMed]
- G. M. Morris, R. Huey, W. Lindstrom, M.F. Sanner, R.K. Belew, et al., AutoDock4 and AutoDockTools4: Automated docking with selective receptor flexibility, J Comput Chem, 30 (2009) 2785-91.
[Crossref] [Google Scholar] [PubMed]
- S. V. Lim, M. B. A. Rahman, B. A. Tejo, Structure-based and ligandbased virtual screening of novel methyltransferase inhibitors of the dengue virus, BMC Bioinform 12 (2011) 1-12.
[Crossref] [Google Scholar] [PubMed]
- M. M. Jaghoori, B. Bleijlevens, S. D. Olabarriaga, 1001 Ways to run AutoDock Vina for virtual Screening, J Comput Aided Mol Des, 30 (2016) 237-49.
[Crossref] [Google Scholar] [PubMed]
- T. Sander, J. Freyss, M. Von Korff, C. Rufener, DataWarrior: An open-source program for chemistry aware data visualization and analysis, J Chem Inf Model, 55 (2015) 460-73.
[Crossref] [Google Scholar] [PubMed]
- N. Yasmen, Indian Medicinal Plants, 12 (2018) 12-24.
[Crossref] [Google Scholar] [PubMed]
- J. L. Wallace, Pathogenesis of NSAID-induced gastroduodenal mucosal injury, Best Pract Res Clin Gastroenterol, 15 (2001) 691-703.
[Crossref] [Google Scholar] [PubMed]
- S. C. Shih, C.W. Chang, Nonsteroidal anti-inflammatory drugrelated gastrointestinal bleeding in the elderly, Int J Gerontol, 1(2007) 40-45.
[Crossref] [Google Scholar] [PubMed]
- U. Rester, From virtuality to reality-Virtual screening in lead discovery and lead optimization: A medicinal chemistry perspective, Curr Opin Drug Discov Devel, 11 (2008) 559-568.
[Crossref] [Google Scholar] [PubMed]
- M. Hay, D. W. Thomas, J. L. Craighead, C. Economides, J. Rosenthal, Clinical development success rates for investigational drugs, Nat Biotechnol, 32 (2014) 40-51.
[Crossref] [Google Scholar] [PubMed]
- J. L. Dahlin, J. Inglese, M. A. Walters, Mitigating risk in academic preclinical drug discovery, Nat Rev Drug Discov, 14 (2015) 279-294.
[Crossref] [Google Scholar] [PubMed]
- F. J. Duffy, M. Devocelle, D. C. Shields, Computational approaches to developing short cyclic peptide modulators of protein-protein interactions, Computational Peptidology, 12 (2015) 241-271.
[Crossref] [Google Scholar] [PubMed]
- A. Daina, O. Michielin, V. Zoete, iLOGP: A simple, robust and efficient description of n-octanol/water partition coefficient for drug design using the GB/SA approach, J Chem Inf Model, 54 (2014) 3284-3301.
[Crossref] [Google Scholar] [PubMed]
Copyright: © 2022 Shaik Aminabee, et al. This is an open access article distributed under the terms of the Creative Commons Attribution License, which permits unrestricted use, distribution, and reproduction in any medium, provided the original work is properly cited.